There’s New Research on the Science of Barefoot Running
A new model reinterprets those pretty graphs that seemed to show minimalist shoes reducing impact forces
It was Daniel Lieberman, the barefoot-running evolutionary biologist from Harvard, who first got me thinking about impact forces. Back in 2010, he and his colleagues published a hugely influential study in Nature that compared the “collision forces” of feet hitting ground for runners with and without shoes while landing on their heel or forefoot. The stark differences in the paper’s force curves provided crucial scientific ballast for the minimalist running movement—but a new study suggests we may have been interpreting those curves all wrong.
The new study, which appears in the Journal of Applied Physiology, comes from Peter Weyand’s biomechanics group at Southern Methodist University, led by his former doctoral student Andrew Udofa, along with Ken Clark (who is now at West Chester University) and physicist Laurence Ryan. By rethinking what running force curves tell us, they’re able to resolve a longstanding mystery about the role of shoe cushioning and potentially offer an accessible and individualized way of testing which shoes are best for you. To understand why, we first need to look back at Lieberman’s curves.
If you ask someone to run on a fancy force-measuring treadmill (which costs several hundred thousand dollars) or force plate installed in the ground, you can get a characteristic curve that shows you how much force the runner is applying vertically to the ground with each step (and conversely, as Isaac Newton figured out, how much force the ground is sending into your body). Here, from Lieberman’s 2010 paper, is a typical example for someone running barefoot and landing on their heel:
The key feature to notice is the little spike on the left side of the curve. That’s your heel slamming into the ground, a split second before the full force of the rest of your body presses into the ground. The dominant view among biomechanists is that it’s not the overall size of the force (which tops out at about 2.4 body weights here) that determines how likely you are to get injured; instead, it’s how quickly the force is applied, otherwise known as the loading rate. Thanks to the mini-spike of the heel strike, the force in this picture climbs very steeply, which is presumably bad.
Now compare how the force looks when you land instead on your forefoot:
The little spike is gone! That means the loading rate is lower, and you’re less likely to get injured. At least, that’s the theory.
In truth, according to the new study, the little spike is still there; it’s just obscured by the bigger one. Back in 2014, Weyand, Clark, and Ryan proposed what they called a “two-mass model” to explain how these sorts of force curves vary under different conditions. In this model, the force curve always consists of two distinct components: a small spike that corresponds to your foot and lower leg smacking into the ground and almost immediately jarring to a halt; and a bigger, slower spike that corresponds to the rest of your body reaching the lowest point of its up-and-down motion. The overall force is simply the sum of those two spikes.
Here’s what looks like:
The curve shown here looks a lot like Lieberman’s heel-strike curve. But in a 2017 study, the SMU group showed that you get a very similar double peak when you look at world-class sprinters—even though they land on their forefoot. That was an important clue that the mini-spike isn’t a function of which part of the foot touches the ground first. Instead, it’s a function of how quickly you’re applying force to the ground, which depends not just on footstrike but also on speed, shoe characteristics, and other factors.
In the new study, the SMU team compared the force data from eight volunteers in four different shoe conditions: barefoot, minimalist (Vibram FiveFinger KSO), thin-soled racing flat (Nike Zoom Waffle Racer VII), and thick-soled running shoe (Asics Gel Cumulus-14). They wanted to explore a longstanding mystery called the “impact force anomaly,” which is that putting more cushioning in shoes doesn’t seem to reduce the loading rate that runners experience—a seemingly counterintuitive result.
Here’s what their data looks like, showing both the mini-spike associated with the lower leg (J1) as well as the overall force that’s the sum of both spikes, for two speeds (~6:40 per mile and ~3:50 per mile). The vertical axis, as before, is force in body weights, and the horizontal axis is time in seconds.
The first thing to notice is that for each speed the rising slope of the overall force curve—the loading rate—is pretty much the same in all conditions. But the lower leg component (J1) does change: the more cushioning they have, the steeper and higher that peak is. So what’s going on?
What the new results suggest is that we’re wired to automatically adjust our biomechanics to keep the overall loading rate roughly the same. In this study, the runners adjusted the angle of their foot strike to control how long that J1 impact took. When barefoot, they landed on their forefoot, which prolongs and softens the landing, with the calf muscles and Achilles acting as a shock absorber. In the thick-soled trainer, the presence of cushioning allowed them to slam down directly on their heel, which led to a sharper J1 curve without changing the overall loading rate.
What’s key here is the timing of that impact peak. If the initial peak is delayed long enough, it effectively disappears into the main peak, as in Lieberman’s 2010 data. But by delaying that peak, it ends up occurring at a point where the other, slower component of force from the rest of the body is much bigger. In other words, you reduce one of the forces but increase the other, and end up with a similar total force.
I realize we’re getting into the biomechanical weeds here, so let’s zoom out to consider what this means. The biggest practical insight: in the new SMU data set, changing your shoes, on its own, doesn’t significantly change your loading rate. Either your shoe cushioning softens the impact, or you adjust your landing to get cushioning from your calf and Achilles. Take your pick, because the end result—at least under these particular conditions—is the same.
That doesn’t mean this system is foolproof. If you head out for a barefoot run and land on your heels—either because that’s what you’re used to from a lifetime of running in shoes, or because, as in the Lieberman experiment, the guy in the lab told you to—then you’re going to generate really high loading rates, because neither protective mechanism (shoe cushioning or forefoot strike) is switched on.
Conversely, if you suddenly switch to a forefoot strike after a lifetime of heel striking, you’ll be putting a lot of unfamiliar stress on your calves as they take on the role of shock absorber. “There likely are injury implications,” Weyand says, “and these do line up with the eager barefoot adapters getting Achilles injuries.”
Weyand is justifiably hesitant to generalize, though. This is a small study of a few volunteers running under very specific conditions at fast speeds. It doesn’t give us the final answers about what shoes or running styles are best for everyone, or exactly how these forces translate into injury risk—but he believes it does give us a better way to answer those questions.
Crucially, the simplicity of the two-mass model means that you no longer need a prohibitively expensive force-measuring treadmill to assess impact forces and loading rates. Instead, all you need to know is how fast your lower leg is moving when it hits the ground; how long your foot stays on the ground; and how long each step takes. You can get those parameters with a high-speed video camera, or these days you could do it with a small leg-mounted accelerometer. Plug them into the two-mass model equation, and it spits out the force curve. So with some fairly simple wearable tech, you should be able to head to a shoe store, try on five pairs of shoes, and know in real time what impact forces and loading rates you generate with each one.
For distance runners, the goal would presumably be to minimize impact forces. But it’s worth noting that the opposite is true for sprinting. The primary way people run faster is by striking the ground harder: the more force you can plow into the ground, the faster you’ll go. “In this regard,” Weyand points out, “minimizing impact and loading rate is directly in conflict with increasing speed.” That’s one reason training shoes and racing shoes are so different—and it’s something else you can test for with this model, trying different shoes and form tweaks to see what maximizes your force output.
If there’s one thing we’ve learned in the decade since Lieberman’s initial results, it’s that we should be cautious about looking at force curves in the lab and assuming we understand how they translate into real-world outcomes like injuries and race times. That applies to the new SMU data too. But the model has the potential to solve two very current problems: taking biomechanics out of the rarefied lab environment and into the real world; and extracting useful insights from the firehose of personalized data generated by emerging wearable tech. Hopefully there will soon be an app for that.
My new book, Endure: Mind, Body, and the Curiously Elastic Limits of Human Performance, with a foreword by Malcolm Gladwell, is now available. For more, join me on Twitter and Facebook, and sign up for the Sweat Science email newsletter.
You May Also Like
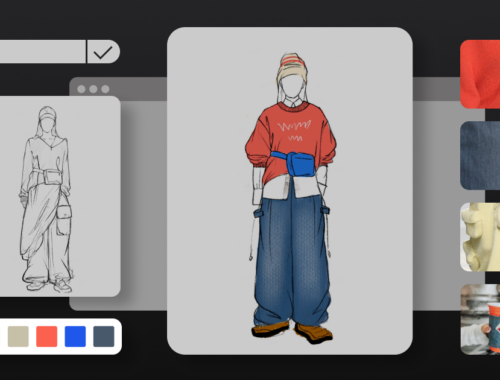
The Future of Fashion: How Artificial Intelligence is Revolutionizing the Industry
February 28, 2025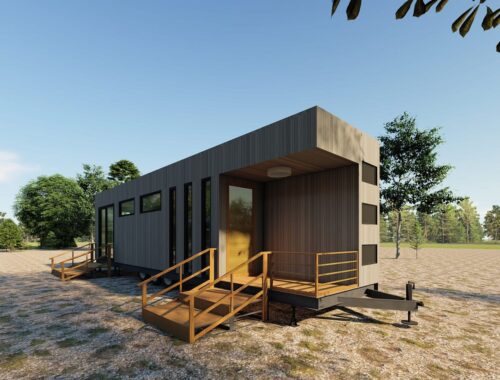
ユニットハウスのメリットとデメリットを徹底解説
March 20, 2025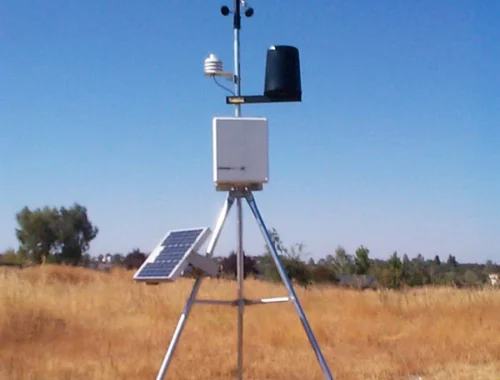